Colonies in Space:
Chapter 12 – The Shell of the Torus Chapter 12 – The Shell of the Torus
Colonies in Space
by T. A. Heppenheimer
Copyright 1977, 2007 by T. A. Heppenheimer, reproduced with permission
Chapter 12 – The Shell of the Torus
It was not a very large meteoroid as these things go, just a small chip from an old burned-out comet, a chunk thirty feet across. It had been knocked away at about the time some apelike creatures in Africa had begun to discover they could live on the ground, down from the trees-15, maybe 20 million years ago. Since then it had been orbiting in space, crossing and recrossing the orbit of Earth, yet never coming very close. Still, this could not go on forever. Someday it would certainly strike the earth, unless it hit Venus.
It was in that part of the orbit where it would begin to draw nearer Earth, feel its attraction a bit more strongly. This was nothing new, it had happened a hundred thousand times before. Space is big and the earth is small, a difficult target to hit with a rock moving randomly through space.
As the weeks progressed it moved closer. Had someone been aboard it, he would have seen the earth grow brighter and then begin to appear as a disk. Off sunward the moon would be distinguishable from the stars and in time it too would show a disk.
The rock was too small to see in even the largest telescopes. But could it have been seen and tracked, astronomers could have computed its orbit and determined where it was heading. Had they done so, they would have noted with some interest that this meteoroid would be passing relatively close by. Its path would be leading it between the earth and moon, almost midway between them, at a speed of three miles per second. The astronomers’ interest would be only casual, however. Other rocks, large enough to see and track, had passed similarly close. There would be no significance to this new one. Within a year there would be other chunks of similar size on similar trajectories.
The navigation room in the space colony near the top of the central hub, adjacent to the docking ports, controlled the small radar on the docking area which scanned surrounding space for incoming craft. There was no need for either the navigation room or radar, but under the Space Standards Act every manned spacecraft was required to have navigational facilities. For the purposes of this act, the Circuit Court of the District of Columbia had ruled that the space colony indeed was a manned spacecraft. There was no permanent officer on duty there and if there was, he probably would have been asleep. So no one saw the thin, light trace on the radar screen as the meteoroid headed in.
It struck the inner rim and passed through, leaving a neat hole. It was a fragile thing, easily broken, and the force of its impact was that of an explosion. It broke into several large chunks as it passed through the wall and these chunks fanned out as they ripped through the interior like fragments of a dumdum shell. When they reached the outer wall of the colony, they struck with the force of several tons of TNT. Where there had been Peachtree Park and the Apple Valley cluster of apartments there now was a hole 300 feet wide.
It took less than a minute for everything else to happen. The air in the colony began to pour through the gash at ten times the speed of a hurricane. For over a mile on either side of the hole, the rushing winds within the colony tore loose all buildings, carried off people and trees. The impact had been so sudden that there was no warning, no hope of warning. The shocks and blasts of the impact traveled round the colony at the speed of sound. But at the same speed came the destructive hurricane–waves of rushing air swirling all into a tumbling, roaring, hurtling mass which gushed through the broken colony and into empty space.
On the side of the colony away from the impact, the winds were not so strong and the destruction less severe. But in less than a minute there was too little air to breathe. No one had time to take action, none could save themselves. Some panicked, rushed outside, and were caught up in the winds. Many mercifully died in the wreckage of buildings. Where the houses did not fall and where people did not panic, there was time to head for a shelter but there were no shelters. Implacably, the air escaped, people gasped a bit, collapsed, their lips blue.
Several days later, the first ship of the Survey arrived. The colony was now surrounded by orbiting floating debris. The captain watched his radar and advanced slowly. Some of the debris drifted close enough to see clearly. There was the trunk of a tree– a palm, perhaps? It was hard to tell, its top had been sheared off. Again, there was what once might have been the roof of an apartment. But what made Captain Freitag shudder in later years, whenever he recalled it, was that other thing. It was a drifting form, limbs stiff, its face tight with horror and surprise–and with its torso cut nearly in two from a collision with some hunk of debris.
Someday, Hollywood will probably make a movie somewhat like this. Something like this may actually happen. The children of science who go forth to colonize space will find it a rich, fruitful medium in which to shape a new civilization. But nature does not yield its gains without risks and there will be hazards in space.
Probably the most frightening space disaster imaginable would be the collision with a swarm of large meteoroids, followed by explosive decompression and death for all aboard. This eventuality will probably only occur in future versions of such movies as Earthquake. The real meteoroid problem is finding minor leaks and repairing them in reasonable fashion.
The horrifying vision of a meteoritic disaster to a colony in many ways resembles the prospect of a nuclear disaster on Earth. Both involve arcane technologies with which few people have had direct experience. In both cases, people’s convictions as to the smallness of the risks must rely heavily upon the testimony of experts, rather than upon the kind of common experience in which we adjust our driving to avoid a road mishap. Either disaster could strike with no warning, wiping out whole populations in a moment. The strange-looking domes of nuclear plants and the constant news reports of minor mishaps continually remind us of nuclear risks. In space, the occasional noticeable meteoroid impact, as well as astronomers’ reports of small objects, will remind colonists of their own dangers.
But there is an important difference. We do not yet have so much or such thorough experience with nuclear plants to say for certain that their safety systems will always work. We can run test after test, pile safeguard upon safeguard. In the end, we largely come down to the fallible judgments of imperfect human beings. In the case of meteoroid dangers, we can see the objects of interest, accumulate statistics on impacts and sizes. We can go into space and measure the rates of impact by various types of meteoroid. What’s more, we can construct a reasonably good understanding of how the probability of being struck depends on the size of the object.
In thinking of a rocky or gravel beach, there is some idea of the distribution of meteoroid sizes. There are a few large rocks and boulders big enough to be worth standing next to and have a picture taken. There are more smaller rocks and a great many smooth round stones or pebbles. More numerous are pieces of gravel. Far outnumbering all are the individual grains of sand.
So it is in space. The few large meteoroids, impressive enough to wind up in museums, fall at the rate of perhaps one a year. But on a clear night, you can go outside to look for shooting stars. You will probably spot at least a few, and each is caused by a meteoroid the size of a small sand grain.
We have data on meteoroid sizes and impact rates not only from observations made on Earth and in nearby space, but from instruments on the moon. These detect the shocks and small moonquakes which result when something hits the moon. The data from all sources tend to agree and give the following picture of meteoroid hazards to the colony:
Size | Mass | Rate of Occurrence | Worst Effects |
1/4-inch pebble | 1/100 oz. | Every 3 yrs. | Loss of window panel. Atmosphere escapes at 1% per hour. |
2-inch rock | 3-1/2 oz. | Every 7,000 yrs. | 60% of atmosphere escapes in 10 hours. |
Boulder | 1 T | Every 250,000,000 yrs. | Severe damage. |
The kind of catastrophe described at the beginning of the chapter probably would not occur before there was an entirely different catastrophe. The sun will expand into a red giant and engulf the earth—perhaps 5 billion years from now.
Anything heavier than a few hundred pounds would produce severe damage when striking the colony, about the same effect as setting off a fair-size bomb in the World Trade Center. However, even meteoroids as small as a pound would be rare and much less of a problem than airliners crashing into backyards on Earth.
The most common meteoritic effect would be a slow sandblasting of the windows and hull produced by micrometeorites. Over several centuries this would reduce the colony’s bright metallic appearance, rendering it dull and gray and the windows would become less transparent, less clear. Once every few years the colony would probably be hit by something large enough to make people take notice. This would break a window, perhaps, or punch a hole in the hull.
On the scale of the colony, this would be similar to an auto puncture by a nail. A nail puncture usually results in a slow leak which you notice a few hours later when you stop for gas. The gas station attendant will cover the tire with soapy water and you will see the bubbles where air is escaping. It takes at least several hours for the tire to go flat, and similarly the leakdown rates in colonies will be quite slow. Daniel Villani of Princeton University has computed that with a hole as large as three feet wide, it would still take over ten hours to lose half the atmosphere of a colony.
Small leaks will be fixed much as you would repair a minor hole in a boat with a patch and caulking compound. For a larger hole, there probably will be something like a large metal tortoise shell which can be set over the hole to stop the leak. Workers in space suits then can weld an appropriate patch into place or replace a broken window pane.
There is a type of artificial meteoroid which may be dangerous to a colony. The mass-catcher described in chapter 6 would be propelled by rotary pellet launchers. The launchers resemble garden sprinklers which throw rocks—they eject small pellets at high velocities to give thrust. The pellets will not disappear but orbit through space as artificial meteoroids. They will not be sand grains, and will be just the right size for breaking windows or punching neat holes in the colony wall.
The pellets will not be ejected in any particular direction, but will be scattered and their hazard will be a matter for statistics. The astronomer George Wetherill, of the Carnegie Institute of Washington, has studied the danger from meteoroids with orbits similar to the pellets. He finds the pellets, like the meteoroid at the beginning of this chapter, will quickly move away from Earth’s vicinity but stay between the orbits of Venus and Mars, crossing Earth’s orbit repeatedly. After 10 to 100 million years, they will be swept up by Earth.
Earth has a billion times more area than a space colony. If we wish to have only one impact from a pellet every ten years, we can eject something like 10 quadrillion (10 followed by 15 zeros) pellets. This is a million times the mass of pellets to be ejected in transporting material for the colony.
The hazard from meteoroids will be very small and the environmental effects of the rotary pellet launcher will be entirely lost in the natural effects due to meteoroids. There is little danger with large particles of space matter, those big enough to hold in the hand. Unfortunately the same is not true of the smallest particles of space matter, the cosmic rays.
Cosmic rays are charged particles which stream outward from the sun and inward from the galaxy. They are predominantly the nuclei of atoms of hydrogen, moving at close to the speed of light, but it is likely that every element of the periodic table is included in their nature.
When the sun is quiet, it emits a solar wind of protons and electrons which stream outward at speeds of 300 miles per second. The particles do not penetrate and pose no threat to the colony. However, every eleven years the sun enters an active phase and can produce solar flares. In these sporadic violent eruptions, the sun emits blasts of high-energy protons which can deliver dangerous doses of radiation. In the flares the particle energies usually are below a billion electron volts, but the worst radiation conditions occur during eruptions similar to the great flare of February 23, 1956. The proton energies then can range up to several billion electron volts, which gives approximately the radiation environment inside a research-type nuclear accelerator. An unprotected human being would need less than an hour to receive many times the fatal dose of radiation.
Cosmic-ray flares occur once every several years, and flares as large as the 1956 event occur every few decades. Because many of their protons travel at nearly the speed of light, there are only a few minutes between detection of the flare by telescopes and the arrival of the worst of the cosmic rays. People not in a sheltered place will have very little time to get to one. Once a flare begins, its most dangerous time lasts for somewhat less than a day, as streams of energetic particles course in all directions.
Cosmic rays are dangerous because, being electrically charged, they break chemical bonds when they pass through tissue. This can create damage to cells which will lead to cancer or damage chromosomes to produce genetic mutations. The damaging power of a cosmic ray is related to its “ionizing power,” which measures how many chemical bonds are broken. Ionizing power does not increase with energy as might be expected. The most ener getic particles, moving close to the speed of light, pass swiftly through the body and do relatively little damage. It is at lower energies, speeds less than the speed of light, that the particles have more time to break bonds and do much greater damage.
If we calculate the annual radiation dose which the general flow of cosmic rays would give to an unshielded person (aside from the occasional solar flare), the dose is about ten rem per year. The rem is a unit of radiation exposure used in the nuclear industry. This is not a high dose. It is only twice the dose for radiation workers recommended by the Atomic Energy Commission for adults working in industries where exposure to radiation is likely to occur. There is evidence that exposures to steady radiation levels up to fifty rem per year will not result in detectable damage. If this total dose were the only problem, then the solution would be a simple matter of providing light shielding over the colony or construction areas, along with heavily shielded shelters to retreat to during the infrequent solar flares. But the problem is more complex.
Included in the cosmic rays are substantial numbers of iron nuclei totally stripped of electrons. When a fully ionized iron nucleus is traveling at less than half the speed of light, its ionizing power is several thousand times that of an ordinary proton. Passage through the body of a single iron nucleus destroys an entire column of cells along its path. The total amount of energy which the particle dumps into the body is small, but it is highly concentrated. This radiation can not only increase the risks of cancer, it can provide pathways of damaged or dead cells along which the cancer can spread and grow.
The iron nuclei do even more. They destroy nerve cells in the brain and spinal column which cannot reproduce themselves. Once the cells are dead, they can never be replaced. Studies of Apollo astronauts indicate that on their two-week lunar voyages, they may have lost as much as one ten-thousandth of their nonreplaceable neurons. Under such conditions after several years in space, the loss could reach several percent. For children, especially, the effects could be devastating.
It is also important to be aware of the phenomenon of secondary particle production. When high-energy particles collide with matter, as in a radiation shield, they give off a spray of particles. These in turn may produce more particles. In the presence of very energetic particles, therefore, a little shielding may cause an even larger radiation dose than if there were no shielding. There also is the possibility that a little shielding will slow down fast iron nuclei, making them more damaging to tissue.
How can we protect the colony against radiation? The first thing is to set a standard. Actually, the U.S. government sets two standards now. For radiation workers—that is, for adults over eighteen—the standard is five rem per year. For the general population, which includes children and pregnant women, the limit is one-half rem per year. This limit is about the level of radiation which occurs naturally in cities of high elevation like Denver. In addition, this standard provides that the colonists will receive no more iron nuclei over thirty years than Apollo astronauts received in two weeks.
To reach these limits the same means of protection which the earth provides can be used. The earth has a magnetic field which deflects many particles and an atmosphere thick enough to shield us from those that get past the magnetism. Both will serve for protecting the people of a space colony.
For the construction shack and the colony’s external work areas, the solution might be to enclose the construction spheres in a magnetic field. When a charged particle passes through a magnetic field its path curves, and an appropriate arrangement of magnetic fields can form a region where particles cannot enter. Not all particles will be deflected this way, however. High-energy particles will curve only slightly and penetrate the shielded region.
The construction workers can receive adequate protection by designing the shield to deflect particles with energies below half a billion electron volts. The particles deflected would include those from most solar flares as well as the worst of the cosmic rays (including the iron nuclei). The shield can be kept light enough for construction spheres of reasonable size not to produce secondary particles to any major degree.
On rare occasions solar flares would burst forth with an intensity that would make a shield inadequate. For those times the construction areas should be equipped with flare shelters made of enclosed modules with thick walls. At first these walls would be filled with a foot of lead and carbon to stop radiation. Later, the valuable carbon could be removed for use in the colony, with lunar rock substituted. Gerry Driggers, who has done much of the work on construction shack studies, has described the Strangelovian life of people in these flare shelters:
Each [shelter] would be equipped with three hundred comfortable reclining seats, reading material, movies, and some food and drink. . . . Stay times could be made practically indefinite with brief excursions for food and water. . . . One square meter has been allocated per man with individual reclining seats provided, each equipped with a foldaway desk. Entertainment would consist of reading matter, movies, and various games which can be played in a small area. The shelter would naturally-double as the primary recreation area for off-duty crew members. [From a paper entitled “A Baseline Construction Station,” presented at the 1975 Princeton Conference.]
However, Dr. Strangelove, of the Stanley Kubrick movie, proposed to have people live under such conditions for ninety-three years, and Dr. Driggers anticipates that “the in frequent use of the shelter will probably be for stays of three to eight hours.”
For the colony itself, all this is insufficient. The exposure limit, one-half rem per year, cannot be attained simply by excluding the low-energy particles and by keeping flare shelters handy just in case. Instead, it is necessary to keep out all particles with energies up to 10 billion electron volts.
There are no more than about half a dozen laboratory accelerators which can produce hard radiation with such energies. The hard-radiation areas of the accelerators are buried under many feet of rock and soil to protect passers-by. To protect the colonists against such particles will be difficult.
It is not possible to use magnetic shielding. The coils of superconducting wire and cable, the cooling apparatus, most of all the structural steel to withstand the magnetic forces—all these together would weigh several million tons. A magnetic shield for the Stanford torus would not be a simple set of coils wrapped around an enclosed space. A shield like this would, indeed, be the colony.
Though magnetic shields will be too heavy, the same may not be true of electric shields. If the colony were to be charged electrically to 10 billion volts, it would repel cosmic rays very effectively. Ten billion volts would repel all particles with energies below the desired cutoff-10 billion electron volts.
While virtually all cosmic rays would avoid the Stanford torus as if it were a leper colony, every electron for hundreds of miles around would feel a strong attraction. They would rush toward the colony with the same high energy, 10 billion electron volts. When they got there, they would do two things, both of them bad. The first would be to produce strong and damaging X rays, which occur when energetic electrons strike a metal surface. The second would be to neutralize the electric charge. The colonists would be no better off than if they had no shield at all. They would actually be worse off, since they would all be dead from the X rays. So if electric shielding is to work, it is necessary to be a bit more clever.
Eric Hannah, Gerry O’Neill’s associate at the 1975 Summer Study, thought of a possible way out. Unlike cosmic rays, electrons are very light and are easily deflected by small magnetic fields. Suppose, he said, we were to build the hub of the colony around a hollow core wound with coils carrying electric current (physicists call such an arrangement a solenoid and often use solenoids to produce magnetic fields); it would produce a field adequate to keep the electrons from reaching the colony. The electrons would all be funneled into the interior of the hollow core where the magnetic field (hopefully!) would keep them from ever reaching the walls. With this arrangement, an electron gun (having roughly the power of the two-mile-long Stanford linear accelerator) could pump out enough electrons to maintain the charge on the colony.
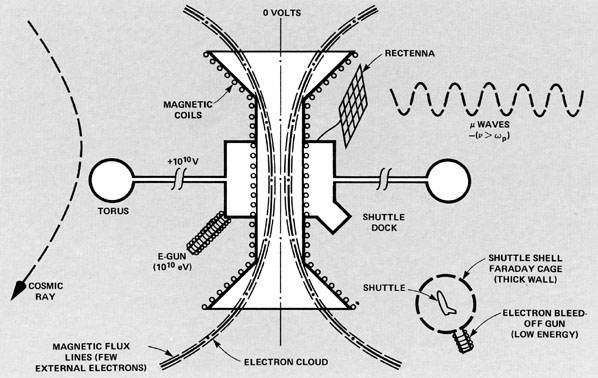
This is the kind of speculative technology that may become attractive once we have learned as much about controlling electrons in free space as we know about controlling electrons in wires. But no one will risk a colony and its people on the off-chance that the electrons in the solenoid will never, never find a way to reach that colony hull to which they are attracted so strongly.
There is an easier way to shield the colony and it is beautifully simple. Just shovel 6 feet of lunar soil around the whole of it. Let the colony hull be six feet under such a covering and all radiation problems will vanish.
The six feet of covering will absorb all cosmic rays as well as most secondary particles. A few of the most energetic particles will produce so many secondaries that some will reach the colony, but there will be no problem with solar flares, even the biggest, and no problem with the iron nuclei in cosmic rays. Babies and mothers will live amid radiation levels no higher than those in Denver or in some of the cities in northern Arizona.
But six feet of soil over all 440 exterior acres of the Stanford torus is a rather large pile. It is 10 million tons, every pound of which will have to be shot from the moon by the mass-driver, then delivered to the colony by the mass-catcher. It is this thick shell, this radiation shield, which will certainly be the most massive single item to construct.
It was late in the 1975 Summer Study when we realized this. For a day or two that August, there was serious doubt that we would be able to accomplish our mandate: “Design a system for the colonization of space.”
But it wasn’t long before this shield began to look feasible, and soon it began to look almost inevitable. For much of the 10 million tons will simply be the slag or waste left over after the metals have been taken from the lunar soil. On Earth, the processing of low-grade ore inevitably produces huge piles of useless tailings, dumped into a lake if possible, a hole in the ground if necessary. In space, the same tailings will not be waste. Some will serve for making glass, some perhaps as a source for oxygen. But most of this material, resembling good beach sand, will be used to fill the radiation shield.
This shield will be a hollow shell of aluminum, fitted around the Stanford torus in the way that a bicycle tire fits around an inflated inner tube. This shell will be filled with the sandy slag or tailings, blown in much as wheat is blown into a grain elevator in the Midwest. When a mass-catcher arrives, a part of the shell will provide a convenient spot to store its hundreds of thousands of tons of lunar ores. The construction workers, building power satellites or new colonies, will draw on these stored reserves. They will process them, take from them the needed metals and glass and oxygen and return the tailings to the shell.
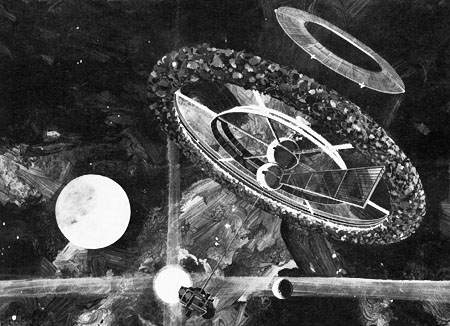
The shell will not be quite the same as a bicycle tire in relation to its inner tube, since it will not rotate. The Stanford torus—the inner tube—will rotate once a minute. But if the shell rotated it would need to be built much more strongly than is reasonable. So it will not rotate, but will enclose the colony hull as the hull rotates past at over 200 miles per hour. There will be a clearance of several feet to keep the hull and shell from touching or scraping. The shell will be tied by cables to the central hub to ensure that no such scraping can occur and the cable lengths will be maintained tight like bicycle spokes. Inevitably, there will be concern that the hull and shell may scrape despite these precautions. The solution will be simple: add more clearance. An extra 4 feet of clearance will only increase the shell mass from 10,000,000 tons to 10,100,000.
How can light get through to the colony under such a shield? The colony mirrors can reflect sunlight down into the Stanford torus. Sunlight cannot be provided by making the shield only a partial shell, leaving a gap. Through the gap would come rays much more penetrating than sunlight. Sunlight, however, reflects off a mirror, but cosmic rays do not. So where sunlight is to come through, we build the shell, not as a solid covering, but as a collection of overlapping V-shaped sections resembling lengths of angle iron. The flanges of these will be the standard six feet thick, with their surfaces covered with mirrors. The sunlight will reflect from mirror to mirror and on inside, but the cosmic rays will be absorbed in the plates of the V’s. The arrangement of these V’s, stacked as they are one above the next, resembles the stripes of a sergeant’s chevrons. It is this “chevron shield” which solves the problem of letting in the light.
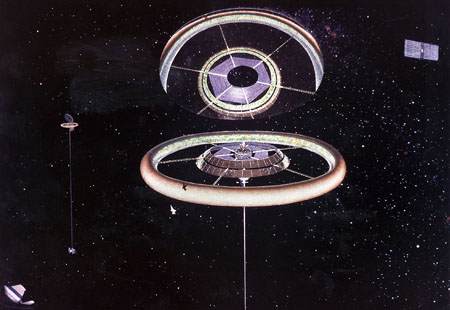
Overall aspect of the space colony. The top structure is a large mirror, in which an image of the colony is seen. A second colony, at the right, shows how the Stanford torus is connected to the extraction facility. A power satellite is at the upper left. (Donald E. Davis painting courtesy NASA) [larger image]
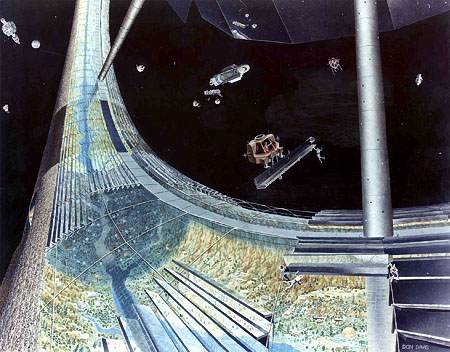
Installation of chevron shielding in the colony. The chevron shields are the structures resembling lengths of angle iron. (Donald E. Davis painting courtesy NASA) [larger image]
When we have protected the colony, we find we have actually recreated the protection we enjoy on Earth. The atmosphere above us gives us the protection we would receive from a shield of lunar soil ten feet thick. The colony’s protection will be a bit less, but will follow the same principle.
Inside this protection the colonists can settle down to build their lives. They can establish schools, organize clubs and churches, in short, do nearly anything they would do back on Earth. Including going to the movies to see a film about a meteoroid hitting a space colony.